Tough Welding — Achieving Required Impact Toughness for Arctic Projects
Both structural- and pipe-welding in the Arctic present challenges for design engineers and fabricators alike. Steel, particularly high strength steel, can become brittle at the temperatures seen in the Arctic environment. Likewise, welding these materials can transform ductile steel into a brittle weldment unless the proper processes, procedures, equipment and filler metals are utilized. Achieving the proper impact toughness — or resistance to cracking — is critical to producing a finished product that meets design requirements and fabrication code minimum requirements.
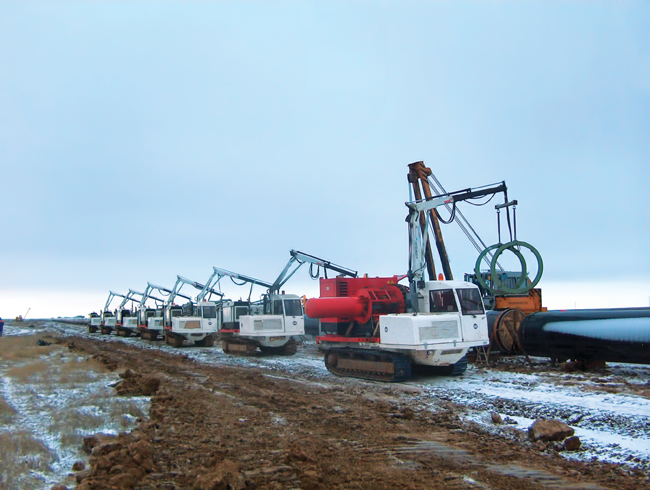
toughness — or resistance to cracking — is critical to producing a
finished product that meets design requirements and fabrication
code minimum requirements.
What is impact toughness and why is it important?
Impact toughness can be defined as “the ability of a metal to deform plastically, and to absorb energy in the process, before fracture.” The Charpy V-Notch (CVN) Impact Toughness Test (ref. ASTM E23) is typically used to measure impact toughness in steel. See Figure 1. It is an important engineering property because tough materials yield or deform prior to failing. Brittle materials fail abruptly without warning, and this often leads to catastrophic equipment damage, injuries to people or the environment, and in the worst cases, loss of life.
Impact toughness in carbon steel generally decreases as the temperature drops below 0 degrees Celsius (32°F). For welded structures or piping intended for service in the Arctic environment, low-temperature impact toughness becomes a serious challenge. Typical design temperatures for cold weather environments fall in the range of -10 degrees Celsius to -30 degrees Celsius (14°F to -22°F) and for Arctic applications, design temperatures start at -40 degrees Celsius (-40°F). Engineers often add a factor of safety by setting the material testing temperature 20 degrees Celsius below the design temperature.
Construction codes specify the minimum impact toughness that base materials and welded joints must achieve at a given temperature. Specific toughness requirements are a function of the materials used, the design temperature for the welded piping or structure, and in some circumstances, the thickness of the material.
Design — selection of filler metals and welding processes
Not surprisingly, filler metal selection for Arctic projects is critical. Filler metals contain many elements, each of which provides distinct characteristics to both the welding process and the completed weld. These elements can include deoxidizers like silicon and manganese. Manganese influences strength and toughness, as well. Chromium increases strength and, in high enough quantities, corrosion resistance in steel alloys. The addition of nickel, and the elimination of phosphorous and sulfur, are particularly helpful in creating good impact toughness.
Nickel helps alter the weld microstructure to increase strength and provide cracking resistance. In some cases, wires with added nickel may have a slightly more sluggish weld puddle and/or generate additional spatter, but the increased toughness generally offsets such disadvantages.
The presence of phosphorous and sulfur, in both the filler and base metals, is especially detrimental to weld toughness. The harmful effects of these elements are amplified in single-pass applications where more of the base metal mixes with the filler metal, increasing the chance for weld metal to pick these elements up from the base material. It is important to select both base and filler metals with as low of a phosphorus and sulfur content as possible. In the case of filler metals, no more than 0.03 percent is recommended.
Filler metal manufacturers control the flux chemistry in filler metals, including shielded metal arc welding (SMAW), flux-cored arc welding (FCAW) and submerged arc welding (SAW) products to influence the nitrogen and oxygen, which is allowed to react with the molten weld puddle to form beneficial nitride and oxide precipitates in the steel. When these precipitates are present in the right size and quantity, they serve as strengthening agents that also might play a beneficial role in improving toughness, particularly in cold weather applications. The effects of these two elements depend greatly on the steel alloy. Allowing nitrogen to diffuse into the weld puddle generally does not benefit low carbon and low alloy steels. However, there are strong benefits gained in some high alloy steels, tool steels and stainless steels through intentional additions of nitrogen to the weld.
There are trace-alloying elements in filler metals that have a strong influence on mechanical properties, including carbide and nitride formers like titanium, vanadium, cobalt, niobium and boron. Some of these elements serve as grain-refining agents — as a general rule, fine-grained microstructures have better lower temperature impact toughness.
When evaluating filler metals’ properties for a project, select those that meet the minimum requirements set forth by code and contract requirements, plus a small margin of safety. Often times the engineer seeks to choose the filler metal that has the highest mechanical properties available. Often this results in trade-off in terms of welding performance and ease of use.
Pre-heat, interpass temperature control and post-weld heat treatment
When welding in an Arctic environment, it is critical to control the cooling rate of a completed weld. This goal is accomplished through control over the pre-heat, inter-pass temperature and the heat input used during welding. Heat input directly affects solidification and microstructure formation, and microstructure (grain refinement) has a strong effect on the impact toughness. Moderate heat input produces a more robust microstructure.
Pre-heating welds is a requirement of most welding codes when the ambient temperature is low, as in onsite welding in an Arctic environment. The benefits of pre-heating are well known: reduced risk of hydrogen-induced cracking, reduced distortion and reduced residual stresses.
Control of interpass temperature while welding has similar benefits but control can be difficult to achieve. Temperatures can fluctuate over a wide range when welding thick materials. On large
weldments, there can be significant localized cooling if a single welding operator is making the weld. By the time he or she finishes one pass, the start point can cool off significantly. If a welding operator is skipping around in order to control distortion, there can also be large swings in the maximum interpass temperature achieved on a large fabrication. If the welding operator or fabrication team is using conventional torches for heating then the skill and attention to detail of the welding operator can have large influence on just how uniform heating is, especially on a large weld or a component with an irregular shape.
The solution to many of these concerns is to switch to newer technology for pre-heating and maintaining weldment interpass temperature. The two choices available are resistance heating and induction heating. Both react to additional heat input from welding by reducing power to the heating coils in order to maintain the temperature set point. However, induction heating offers a greater level of convenient, speed and efficiency.
An important benefit to induction heating is the improvement in workplace safety. Induction heating eliminates the open flame in the workplace, as well as the need to store flammable gas cylinders in the welding environment, thereby lessening risks. The process is also more comfortable for welding operators, as it delivers heat to the steel, not the welding environment. The result is less stress and discomfort to welding operators, and can help improve the quality of the work being completed.
Conclusion
Careful control of the microstructures that form during weld solidification, cooling and through any post-weld heat treatment (PWHT) are critical to achieving and maximizing low temperature impact toughness for Arctic applications. As steel strength levels rise, control of weld microstructure determines the impact toughness of a weld.
The welding engineer who creates a welding procedure, and the welder who executes it, have control over these outcomes. The former establishes the limits for heat input during welding, the pre-heat and inter-pass temperature, and any PWHT. The latter exerts even greater control by carefully following the welding procedure to gain the best results. As with any application, when in doubt about selecting filler metals or equipment for a low temperature application, consult a trusted manufacturer or distributor for assistance.